Microbiome in health and disease
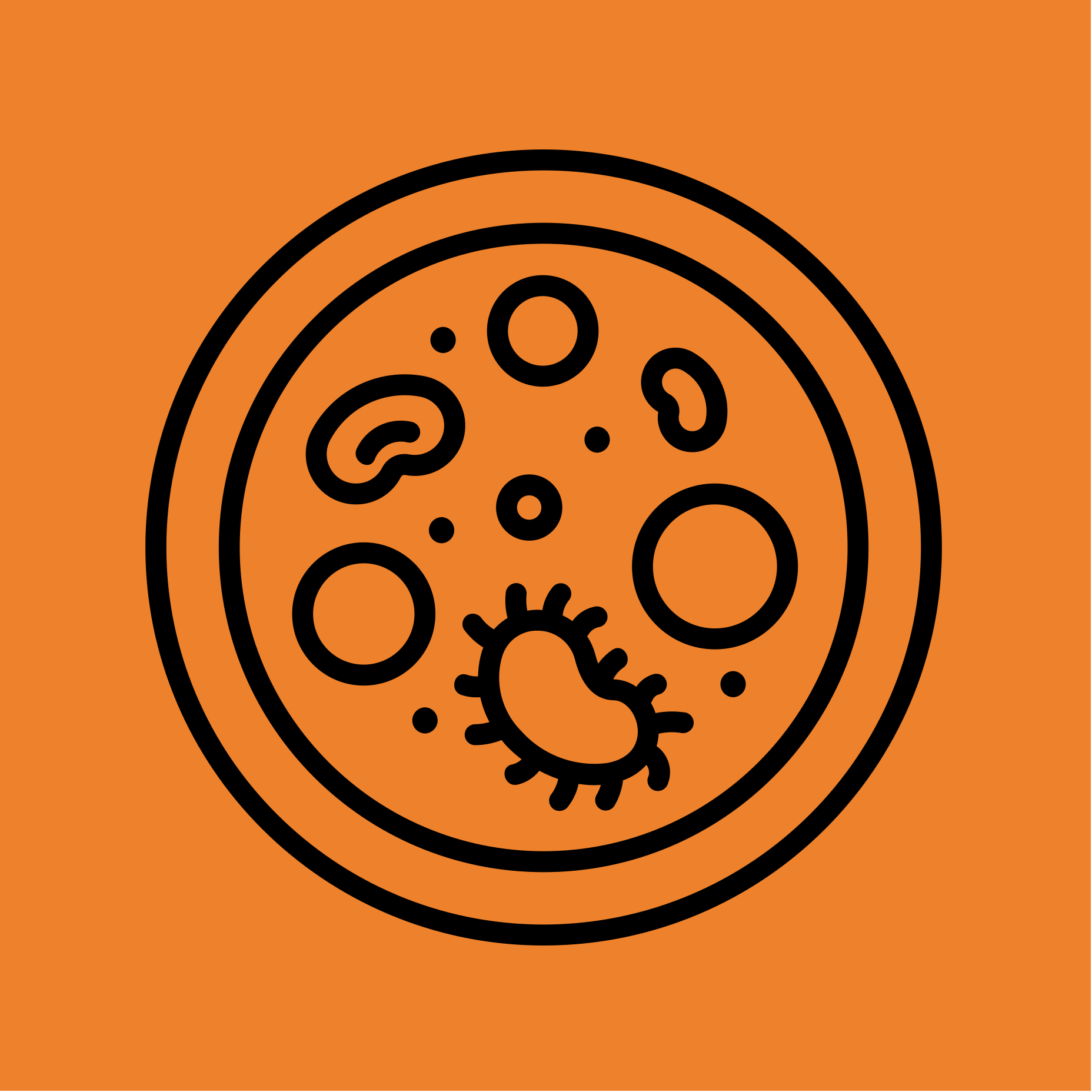
The emergence of metagenomics has profoundly transformed our understanding of complex bacterial communities (microbiomes). In general, such studies rely on the use of sequence surveys, based for example on 16S rRNA sequences, which detail the composition of the microbiome in terms of species, but provide only limited functional insights. Moving beyond such surveys, our lab has pioneered the use of metatranscriptomes to functionally interrogate microbiomes (Jiang et al., 2016) requiring the development of dedicated analytical pipelines such as MetaPro (Taj et al., 2023). Using this technology, we showed how host genotype can modulate microbiome expression without altering community structure, an important discovery that emphasizes the need for more functionally focused studies of the microbiome (Xiong et al., 2017). These studies have been complemented with the development of a new diagnostic to survey the eukaryotic components of the microbiome (Popovic et al., 2018).
In ongoing work we are applying these technologies to reveal the contribution of microbiota to a host of diseases including IBD and global health projects focused on malnutrition (Popovic et al., 2021).
Parasite genomics
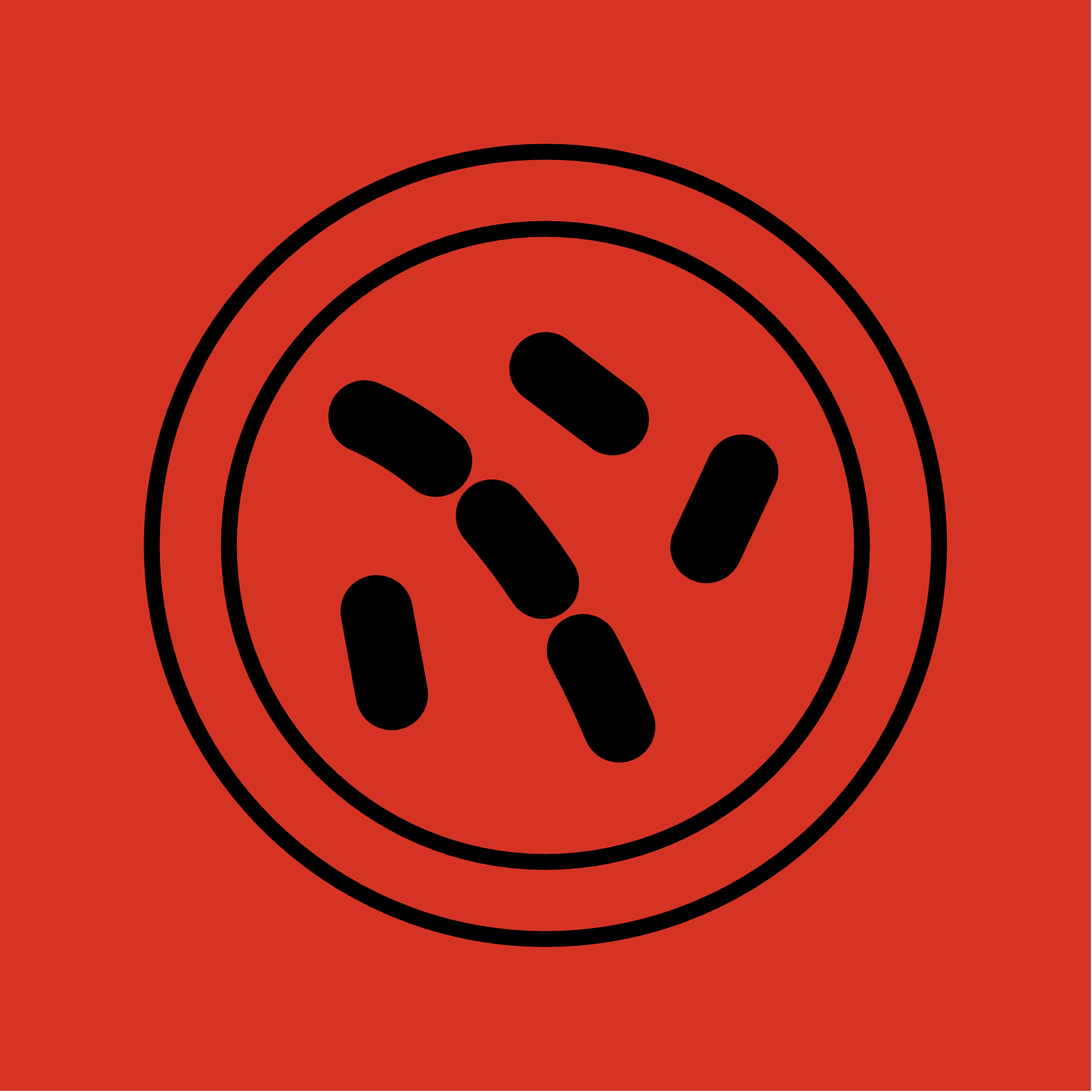
Parasites are a global health burden. Plasmodium affects 300 million people annually, killing 1 million, Toxoplasma, is life-threatening in pregnant women and the immunocompromised, while parasitic worms cause devastation and misery to billions worldwide. Despite their significance, few drugs are available and resistance is emerging to front line therapeutics. To lay the foundation for new drug development strategies, our lab has been involved in a number of international genome sequencing initiatives focusing on organisms that impact global health (Parkinson et al., 2004; Tsai et al., 2013; Lorenzi et al., 2016; Cotton et al., 2016).
The generation of these genome resources help hundreds of research groups prioritize genes for detailed molecular characterization prior to drug and/or vaccine development. Recently our lab led an international consortium to study the genome of the protozoan parasite, Sarcocystis neurona, a member of the most successful protozoan parasite genus on the planet (Blazejewski et al., 2015). This study revealed a number of molecular innovations that support its transition from a purely enteric parasite dependent on a single host, to one that is capable of invading the tissues of multiple intermediate hosts. To further aid the identification of genetic variants that impact pathogenesis, our lab developed an innovative genetic analysis tool, PopNet that uses networks to visualize population structure (Zhang et al., 2017).
Our current investigations focus on applying these tools and approaches to understand the emergence of the human pathogen Neisseria gonorrhea as a global ‘superbug’.
Systems biology of infectious disease
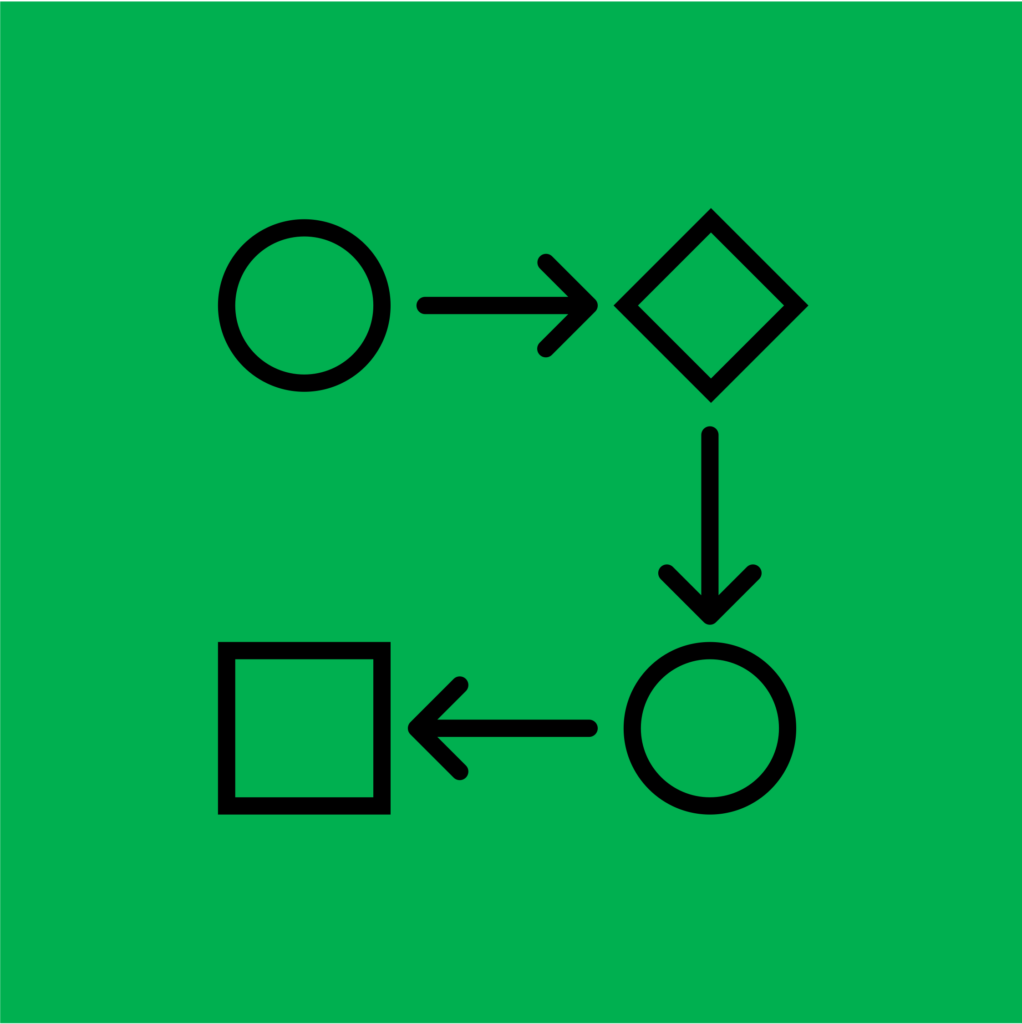
With the availability of increasing numbers of pathogen genomes, the key challenge is interpreting these rich datasets to understand how they are able to infect and cause disease. Our lab applies population and systems biology approaches to identify the genes and pathways that contribute to pathogenesis. For example, we have used comparative genomic approaches to classify arsenals of virulence factors, such as Toxoplasma surface proteins (Wasmuth et al. 2012) or bacterial biofilm machineries (Bundalovic-Torma et al., 2020).
Due to their essential role in driving growth, a major focus of our systems analyses centers on metabolism. Applying bioinformatics tools developed in house (Hung et al., 2010; Nursimulu et al., 2022), we have constructed metabolic models for a variety of pathogens. These models are then used in computer simulations to predict the impact of gene knockouts or changes in available nutrients. For example, applying flux balance analysis (FBA), we showed that growth of normal and hyper-virulent strains of Toxoplasma is driven by differential regulation of energy pathways (Song et al., 2013). We also identified enzymes encoded by the bacterial symbiont of the filarial worm, Brugia malayi that provide essential functions to its host, targets that were validated in drug inhibition assays (Curran et al., 2020).
These studies are currently being extended to investigate how metabolic interactions impact the dynamics of complex microbial communities.
Biological network analysis
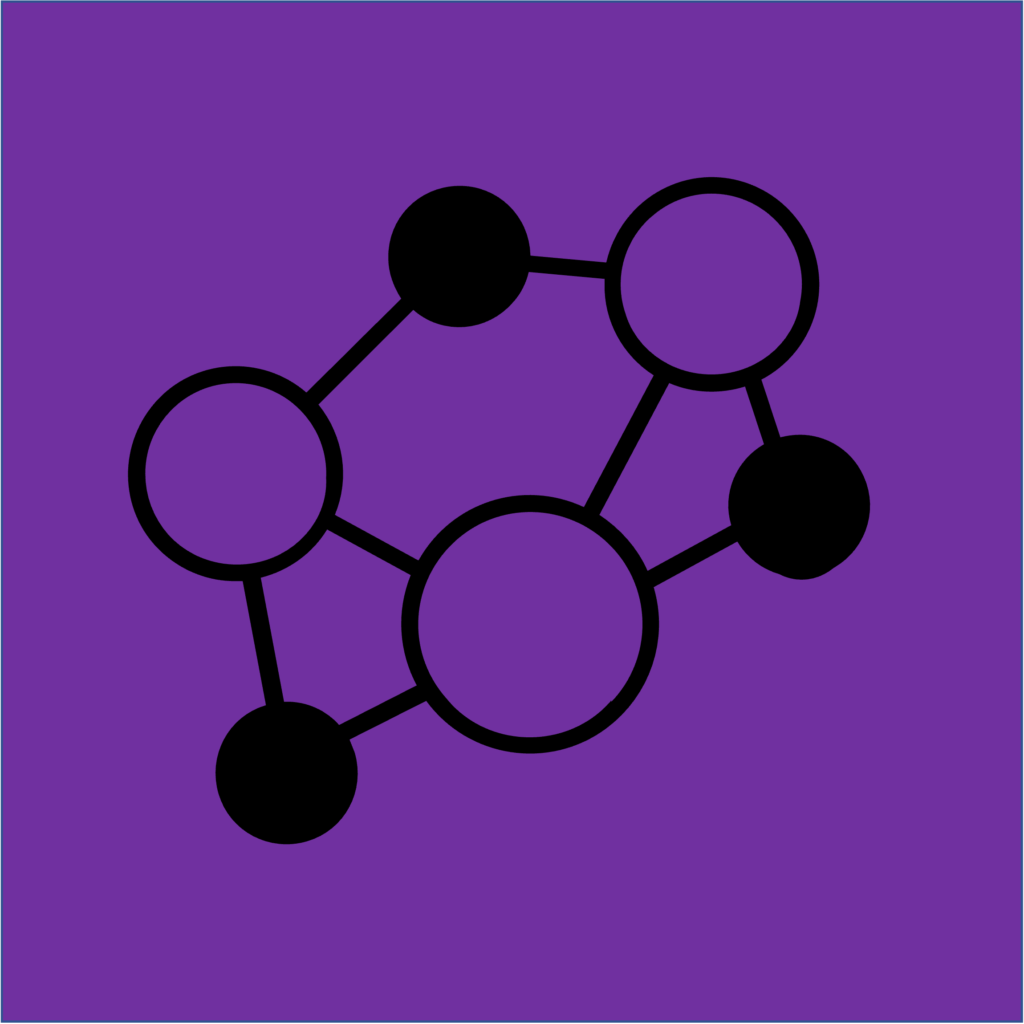
Genes and their protein products do not operate in isolation but form components of intricate biological systems such as protein complexes and biochemical pathways. Characterizing these relationships is critical if we are to understand how these systems function. In collaboration with researchers at the University of Toronto, our lab has generated and analysed the first large scale protein interaction networks for yeast, E. coli and metazoans (Krogan et al., 2006; Peregrin-Alvarez et al., 2009; Wan et al., 2015; Babu et al., 2018). These data represent powerful community resources that have seen widespread adoption across the field (our yeast protein-protein interaction study for example has received over 2000 cites). In addition to providing insights into the organization and evolution of interaction networks, these data provide scaffolds to better interpret orthogonal ‘omics datasets.
Currently we are applying mass-spec based technologies to understand the organization of complexes that help drive the malaria life cycle as well as identify host targets of proteins secreted by intracellular parasites.
Our work is funded by a number of granting agencies including the Canadian Institutes for Health Research, the Natural Sciences and Engineering Research Council of Canada, the National Institutes of Health and the Ontario Ministry of Research and Innovation.